A constant preoccupation of the Lagide kings, pressured by their politics of prestige and expansion, was to increase agricultural productivity. Each region (or nome) is under the responsibility of an economist (the Greek name is Oikonomos), charged, among many other tasks, to “control the delivery canals across the fields, from which the peasants draw water conveyed to their cultivated fields; to verify that the feeder canals have the prescribed depth, and that their interior space is sufficient.”[183] Every retention basin has its irrigation controller (catasporeos) responsible for water distribution.
Under Ptolemy II, the region of Fayoum, already made productive during the era of the pharaohs, is brought under a new development policy. In Chapter 3, we have discussed the legendary Lake Moeris, and the works realized since the time of the pharaohs (Figures 3.6 and 5.11). The capital of the region, the ancient Shedet of the Egyptians that the Greeks called Crocodilopolis,[184] is renamed Arisone, again in honor of the queen. The ancient hydraulic systems are renovated, new projects undertaken, and the lake level lowered. A chief engineer named Cleon is charged with management of the new developments of Fayoum.
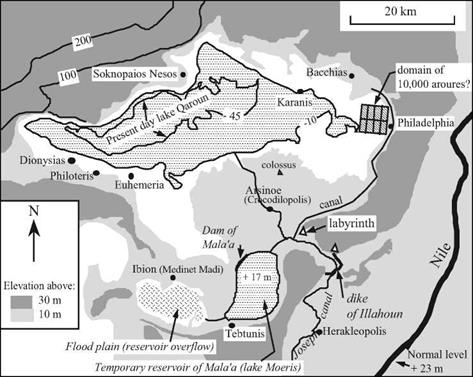
Figure 5.11. Fayoum in the Hellenistic era. We have shown the hydraulic works identified by Garbrecht and Jaritz (1992), and shown the level of Qaroun Lake at the elevation -10 m.23 The level reached by the Nile in flood is about 30 m.
|
Thus, when the minister of the Treasury, Apollonius,[185] [186] receives from the king Ptolemy II Philadelph a concession for the development of a vast domain of 2,700 hectares in Fayoum, he relies on the services of Cleon to establish the economic planning and the layout of the hydraulic infrastructure (Figure 5.12). The correspondence of a certain Zenon of Caunos, who is successively secretary for Apollonius then manager of this domain, gives us a vivid illustration of certain aspects of water resource management in the region. In 258 BC Apollonius has the upper zone of the basin, to the northeast, developed as the “domain of ten thousand aroures”. A new city called Philadelphia is founded nearby. Certain conflicts of interest were inevitable between Cleon the engineer and Zenon the exploiter, as evidenced in the correspondence of Zenon:
“Zenon to Cleon, greetings! The water in the canal has not risen more than a cubit, so the
land cannot be watered from it. Therefore you would be well advised to open the gates to water the land. Stay well! (October 258 BC)
“Panakestor to Cleon, greetings! We sent you a letter on the 19th, asking you to provide us with a team to do maintenance on the bends of the small canal. Well, it seems that you have left us aside in going toward the Small Lake. Instead of avoiding us as you have done, your duty was to meet with us briefly, and having seen for yourself that the land is not being watered, to ask yourself why. Your job is not only to direct the infrastructure works in the region of the Small Lake, but also here. So, at least come meet us tomorrow at the lock and sketch out for us the path canal bends should take, for we do not have this experience. We will provide you with the labor and other facilities, whatever you command. But if you do not come, we will be obliged to write to Apollonios that his land is the only land not to be irrigated in the region of the Lake. So, we are more than ready to make all needed facilities available for you. Stay well. (October 257 BC).[187]
Is it possible that the “Small Lake” in this letter is the reservoir of Mala’a (the lake Moeris of Strabo) to the southeast of Fayoum? This seems to be the chief engineer’s main preoccupation, to the point that he neglects the domain of the minister. It is indeed thought that the works controlling this lake, the dam of Mala’a in particular, were constructed at about this time.[188] The “domain of 10,000 aroures”, to the northeast, is irrigated by a derivation from the Joseph canal (or Bahr Youssouf), the derivation works quite probably located near the Labyrinth. This domain includes parcels that are located on higher ground compared to the other cultivated land of the region. The lower lands must be irrigated first, which causes friction with the villagers outside the domain who think that their water has been confiscated:
“Psenemous to Zenon, greetings! The outlying peasants have taken out their (mules and shovels) and opened the irrigation ditches at the ends of the ten thousand aroures. People from Philadelphia attacked them, (chasing away) the mules and breaking (the shovels). I sent Pelois, son of Pachos, to (tell you of this). But I presume that you already know of these ugly incidents. In order that this business be cleared up as soon as possible, you would do well to order that (their land) be supplied with water. [.. .][189]
Thanks to irrigation, numerous new fruits are adapted and cultivated in Fayoum: olives, pears, apricots, figs, etc. In addition, during this period an attempt is made to develop grains yielding two annual harvests: the first planted at the falling flood, in October, and the second irrigated artificially. This is the sense of a written instruction send to Zenon:
“Apollonius to Zenon, greetings! The King has ordered us to cultivate the land a second time. Therefore, as soon as you have harvested the early grain, quickly water the land by hand. In case this is not possible, install as many irrigation machines as you can, but do not leave water on the land more than five days. Then dry the land and as quickly as possible plant the three-month wheat. Write to me personally when you are ready to harvest the
grain. Go well” (December 256 BC).[190]
The “irrigation machines” of this letter are perhaps balance beams (shadufs), or perhaps the very first models of manual waterwheels that appear in the 3rd century BC.
Two centuries after these accounts, at the beginning of the Roman domination around 25 BC, Strabo sojourns in Egypt and travels up the Nile to Aswan, in the company of the prefect, newly named by Augustus. Twice in his accounts he speaks of irrigation by “machines” (always powered by men or animals) and, in particular, the use of
Figure 5.12 Plans for development of the “domain of 10,000 aurores” and reproduction of a descriptive sketch (Orrieux, 1983; Burkhalter, 1992)
Archimedes Screws and wheels; first in the region of Memphis, then in the region of Aswan.
“There is a ridge extending from the encampment (the cantonment of a Roman legion) even as far as the Nile, on which the water is conducted up from the river by wheels and screws; and one hundred and fifty prisoners are employed in the work; and from here one can clearly see the pyramids.. ,.[191]
“The Nile has very many islands scattered along its course, of which some are wholly cov-
ered at its risings and others only partly; but the exceedingly high parts of the latter are irri – 30
gated by means of screws.”